Deep Heat Treatment: Principles, Uses, And More [Guide]
Are you overlooking a pivotal technique that could revolutionize the performance and longevity of your materials? Absolutely, you are if you're not leveraging the power of deep heat treatment, a process that can fundamentally alter material properties to meet the most demanding engineering challenges.
This advanced thermal treatment method hinges on the precise orchestration of temperature and time to elicit specific material transformations. Imagine a scenario where high temperature and sustained exposure converge, resulting in profound changes in the physical and, at times, chemical properties of the material. The outcomes are transformative: enhanced strength, altered conductivity, and improved resistance to environmental factors. The applications are extensive, reaching from the heart of metallurgical processes in manufacturing to the cutting edge of specialized food preservation techniques. This is the power of deep heat treatment.
The essence of these specialized thermal treatments lies in their unparalleled ability to reshape materials, thus boosting performance, amplifying durability, and fortifying safety. Consider this methodology as the architect behind materials with specific and meticulously controlled properties, indispensable in fields spanning from aerospace and engineering to food science and medical technology. The history of heat treatment is rich with groundbreaking discoveries and pivotal innovations across diverse industries, each building upon the understanding of how heat can fundamentally alter the world around us.
- What Makes 5 Movierulz Kannada 2024 So Hyped Must Know
- Unveiling Shyna Khatri Web Series Popularity Impact Future
This discussion is designed as a springboard into the intricate world of this particular thermal treatment technique and its expansive reach across a spectrum of disciplines.
Understanding deep heat treatment demands a close examination of its foundational elements, each dictating the process's efficacy and application.
- Temperature Control
- Time Duration
- Material Properties
- Process Design
- Equipment Calibration
- Quality Assurance
Temperature control is not merely important; it's critical. Precise temperature profiles are the driving force behind the desired structural changes. Time duration, inextricably linked with temperature, is equally crucial, impacting the depth and uniformity of the material's transformation. Material properties guide the treatment's effectiveness and the parameters required for success. Process design ensures the creation of optimized conditions and predictable outcomes. Equipment calibration is essential to maintain accuracy in temperature profiles, ensuring the process unfolds as intended. Finally, quality assurance protocols provide the scaffolding needed to deliver consistent and reliable results. Envision, for instance, meticulously controlled heating cycles within a furnace, guided by calibrated temperature sensors, producing reproducible outcomes. These outcomes result in materials suitable for highly demanding applications, such as specialized alloys for critical aerospace components. These intricate connections between key aspects underscore the complexity and overriding importance of deep heat treatment in contemporary materials science.
Precise temperature control stands as the bedrock of effective deep heat treatment processes. The ability to maintain precise temperature profiles during these treatments is critical for achieving the desired material alterations and ensuring consistent results. Deviations from these tightly controlled temperatures can lead to a cascade of undesirable outcomes, impacting material properties, potentially compromising structural integrity, and, in the worst cases, rendering the entire process ineffective or even harmful.
- Gradients and Profiles
Deep heat treatment frequently necessitates the creation and meticulous maintenance of specific temperature gradients within the material being treated. This involves the careful design of both heating and cooling cycles, ensuring uniform heating throughout the component or part. Uniformity is not merely desirable; it's critical to prevent the formation of localized stresses and ensure that the desired transformation occurs consistently throughout the material. In the specific context of heat treating steel alloys, for example, carefully designed temperature gradients can produce desired hardness distributions, directly influencing the material's strength and resistance to wear over its lifespan.
- Rate of Heating and Cooling
The rate at which a material is heated and cooled exerts a significant influence on the final microstructure and resultant properties. Rapid heating and cooling, commonly referred to as quenching, can lead to drastically different outcomes compared to slower, more controlled heating and cooling cycles. Variations in these rates can give rise to different metallurgical phases, each affecting critical material properties such as hardness, ductility, and overall resilience. Controlling these rates becomes paramount to ensuring consistency in the final product and avoiding the emergence of undesirable phase transformations that could compromise performance.
- Instrumentation and Calibration
Accurate measurement and control of temperature throughout the deep heat treatment process is nothing short of essential. Sophisticated instrumentation, such as thermocouples and temperature sensors embedded in or strategically placed adjacent to the material, enables real-time monitoring, ensuring that the process faithfully maintains the desired profile. The calibration of this equipment is absolutely crucial to avoid errors and maintain accuracy over time. Inaccurate temperature control translates directly into material properties that fall far short of design specifications, potentially leading to catastrophic failures in critical applications.
- Material-Specific Requirements
It's a fundamental truth that different materials exhibit vastly different responses to temperature changes. Therefore, the selection of appropriate heating and cooling protocols must be carefully considered, taking into account the specific material's unique characteristics. This includes a thorough understanding of its thermal conductivity, the points at which phase transformations occur, and any other relevant material properties. This careful consideration ensures that the heat treatment does not inadvertently introduce harmful or unpredictable changes to the material. For example, different steel types require markedly distinct heating and cooling schedules to achieve optimal results and maintain their desired performance characteristics.
Effective deep heat treatment hinges on precise temperature control, and these facets from the creation and maintenance of gradients to meticulous rate control, accurate instrumentation, and comprehensive material considerations ensure that the process yields the desired outcomes. This, in turn, contributes directly to the reliability and long-term performance of the finished product in a wide range of applications, spanning from structural components in buildings and bridges to high-performance machinery operating under extreme conditions.
Time duration stands as an integral component of deep heat treatment processes, exerting a direct influence on the effectiveness of the treatment and the ultimate outcomes achieved. Prolonged exposure to high temperatures, carefully controlled and monitored, is often necessary to bring about the desired structural alterations within the material. The duration of this exposure has a direct impact on both the depth and the uniformity of the transformation taking place. Insufficient time may result in an incomplete alteration of the material's properties, while excessive time can lead to unwanted degradation or distortion of the material's original form. The relationship between time and temperature during heat treatment is not a simple, linear one; rather, it requires meticulous consideration to optimize the material's resulting properties and ensure its suitability for its intended application.
Real-world examples vividly highlight the crucial role that time duration plays in deep heat treatment. In steel metallurgy, a specific annealing process demands a precise heating time to effectively relieve internal stresses within the metal and achieve the desired level of ductility for subsequent forming or machining operations. Similarly, in the intricate world of semiconductor manufacturing, precise time durations for diffusion processes allow for the creation of carefully controlled doping profiles, essential for the proper functioning of electronic devices. In the realm of food preservation, controlled heating times in retort processes ensure safe and prolonged shelf life by eliminating harmful microorganisms. All of these examples underscore the importance of meticulously calibrated time parameters in achieving the desired effects in deep heat treatments. The optimal time depends intricately on the material's inherent properties, the specific characteristics being targeted, and the capabilities of the equipment used in the process. Variation in time can lead to significant changes in the final material structure and its properties, ultimately impacting the performance, reliability, and safety of the end product. For instance, excessive time at high temperatures may lead to undesirable grain growth in metals, reducing their overall strength and making them more susceptible to failure under stress.
A deep understanding of the intricate relationship between time and temperature in deep heat treatment is vital for achieving consistent and predictable results. It forms a foundational principle in various industries, ensuring material quality, longevity, and safety across a wide spectrum of applications. Optimizing time duration enables the creation of consistent material properties, leading to predictable performance in diverse applications, from the demanding environments of high-performance machinery to the everyday products that enhance our lives. The careful manipulation of time during deep heat treatment remains a central aspect of materials science and engineering across numerous fields, and its importance will only continue to grow as we push the boundaries of material performance and reliability.
Material properties are inextricably linked to the efficacy and outcomes of deep heat treatment. The inherent nature of a materialits composition, structure, and intrinsic characteristicsdictates the parameters that are most appropriate for successful deep heat treatment. The selection of the most suitable treatment processes hinges directly on these underlying characteristics. Consider the following crucial aspects:
The material's thermal conductivity exerts a direct influence on the rate at which heat penetrates the material during the treatment process. Materials with high thermal conductivity necessitate faster heating and cooling rates compared to those with low conductivity. This factor directly determines the rate of temperature change within the material, which in turn significantly impacts the resultant microstructure and, consequently, the material's mechanical properties. For instance, aluminum alloys, which are known for their high thermal conductivity, require different heating and cooling profiles than stainless steel to achieve the desired combination of strength, ductility, and corrosion resistance. Metals with high thermal conductivity, such as copper, allow for faster heat transfer, enabling more rapid heating and cooling cycles and facilitating deeper penetrations of heat treatment. Conversely, materials with lower conductivity may require more time to achieve uniform heating and cooling throughout their volume. This in-depth understanding is essential for achieving the desired properties and avoiding unwanted distortions or any form of material damage.
A material's chemical composition significantly impacts the transformation temperatures and phases that occur during deep heat treatment. Different elemental constituents react differently to heat, and these reactions exert a powerful influence on the formation of specific microstructures within the material. Understanding these reactions is vital for controlling the final properties of the material and tailoring them to the specific requirements of its intended application. For instance, the addition of alloying elements to steel dramatically affects its transformation temperatures and mechanical properties during heat treatment, allowing the development of steels with precisely controlled strength, hardness, and ductility. Specific compositional features, such as carbon content in steel, dictate the optimal heat treatment procedures that must be followed to achieve the desired results. This deep understanding of composition is fundamental for producing materials with precisely tailored performance characteristics.
The initial microstructure of the material exerts a profound effect on its response to deep heat treatment. Existing grain size, crystal orientation, and the concentration of defects within the material will all influence how it reacts to the application of heat and subsequent cooling. This relationship is particularly important in processes where the objective is to achieve grain refinement or recrystallization, as these microstructural changes are directly related to the final properties of the material. For example, a material with coarse grains may exhibit reduced strength compared to a material with fine grains after undergoing deep heat treatment. The initial state of the material forms a critical starting point for the deep heat treatment process, demanding an in-depth understanding and meticulous control throughout. This intricate connection between material properties and deep heat treatment underscores the critical need for careful material selection and comprehensive process optimization to achieve the desired outcomes.
Process design is a crucial element in deep heat treatment, encompassing the meticulous planning and execution of the entire thermal process. It entails defining the precise parameterstemperature profiles, heating and cooling rates, time durations, and the strategic positioning of the material within the processing apparatusto achieve the desired structural and compositional modifications within the material. Effective process design ensures consistency and repeatability, guaranteeing a predictable outcome, which is of paramount importance for industrial applications where reliability and uniformity are essential. This design process is not simply an afterthought; rather, it forms the cornerstone of the deep heat treatment process, requiring careful consideration of numerous factors to optimize the final product and ensure it meets the required specifications.
Real-world examples of sophisticated process design in deep heat treatment are abundant across various industries. In the aerospace industry, where the stakes are exceptionally high, precise heat treatments are crucial to achieving the optimal combination of strength and weight in metallic components used in aircraft and spacecraft. The design must account for the specific material composition, the intended application of the component, and the dimensional tolerances that must be maintained. Similarly, in semiconductor manufacturing, carefully controlled thermal cycles are essential for dopant diffusion and crystal structure refinement, processes that are critical to the performance of microchips and other electronic devices. The process design must precisely manage temperature gradients to avoid damage to the delicate semiconductor materials and maintain uniform doping throughout the material. This illustrates that well-defined process designs are essential for achieving the desired material characteristics in a variety of industrial sectors, where the properties of materials can have a direct impact on performance, safety, and reliability. The intricacy of process design in deep heat treatment demonstrates the precision required for the successful application of this type of thermal processing.
The significance of process design in deep heat treatment cannot be overstated. It ensures consistency, reliability, and repeatability in producing high-quality material transformations, resulting in materials with tailored properties. This systematic approach allows for the standardization of procedures, leading to improved efficiency and reduced waste, which is essential for cost-effective manufacturing. Process design is critical not only for producing high-quality components but also for meeting stringent quality control and safety standards that are often mandated by regulatory agencies. Without a robust process design, achieving the desired outcome in deep heat treatment and obtaining materials with tailored properties becomes an exercise in chance rather than a controlled, predictable method. Understanding and implementing effective process design is thus paramount for modern manufacturing and various applications, ensuring consistency, reliability, and the production of the desired materials that are essential for innovation and technological advancement. The implications extend to the overall economic feasibility and reliability of industrial processes that utilize deep heat treatment, making it a key factor in driving competitiveness and economic growth.
Accurate equipment calibration is indispensable in deep heat treatment processes. Precise temperature control is fundamental to achieving the desired material transformations, and calibration ensures the accurate measurement and control of temperatures during both heating and cooling cycles. Deviations from calibrated readings can lead to inaccurate temperature profiles, resulting in undesirable microstructures, compromised material properties, and, ultimately, defective components that may fail prematurely in service. Consistent calibration ensures the reliability and repeatability of the process, which is a cornerstone of quality control and essential for maintaining consistent product quality.
Consider a scenario in a steel forging facility where variations in furnace temperature readings, stemming from inaccurate calibration, can lead to inconsistent hardness and strength in the final forged parts. This can manifest as brittle components that are prone to fracture under stress, or excessively soft parts that are susceptible to deformation under load, both of which can have serious consequences for the safety and reliability of the products in which these components are used. In semiconductor manufacturing, precise thermal cycles are critical for doping and crystal growth, processes that determine the electrical characteristics of semiconductor devices. Inaccurate temperature control, arising from faulty calibration of the heating and cooling equipment, can lead to non-uniform doping profiles and flawed semiconductor wafers, resulting in devices that do not meet performance specifications. Inadequate calibration can impact the yield of usable products and increase production costs due to the need for rework or scrap. Similarly, in food processing applications, accurate calibration of retort equipment is paramount for safety and preservation. Inaccurate temperature readings can result in insufficient sterilization, leading to potential health risks from contamination or inadequate preservation, rendering products unusable and potentially causing illness or even death.
Proper equipment calibration is not a peripheral consideration but an essential component of deep heat treatment processes. Maintaining the accuracy of measuring instruments ensures the reliability and consistency of results, which is crucial for producing high-quality materials with consistent properties. Consistent calibration prevents defects in the treated material, ensuring the quality and safety of the end product and reducing the risk of failures in service. The consequences of neglecting calibration extend beyond simply inconsistent outcomes; they can lead to substantial production issues, safety concerns, and, ultimately, economic losses. Thus, diligent equipment calibration is crucial for the effective and safe application of deep heat treatment procedures across diverse industrial sectors, and its importance cannot be overstated.
Quality assurance (QA) is an integral aspect of deep heat treatment processes. Maintaining consistent and predictable outcomes is of paramount importance for ensuring the reliability and safety of the materials being treated. QA procedures ensure the integrity and reliability of the heat-treated material, verifying that the process meets predetermined standards and specifications. Defects in the heat treatment process can lead to material failure, safety issues, and economic losses, all of which can have significant consequences. Robust QA protocols are therefore critical for minimizing these risks and ensuring that the treated materials meet the required performance criteria.
- Monitoring and Measurement
Continuous monitoring of critical parameters during heat treatment is fundamental to ensuring that the process is running correctly and that the desired outcomes are being achieved. This includes real-time tracking of temperature, time, and atmosphere conditions within the processing chamber. Sophisticated sensors and data acquisition systems provide precise measurements of these parameters, ensuring that the process adheres to established protocols. Deviations from set parameters are immediately flagged, allowing for prompt adjustments or process intervention to prevent potential issues. This proactive approach helps maintain consistency and mitigate potential problems before they can escalate. For example, in a steel heat-treating facility, continuous monitoring of furnace temperature is crucial for consistent material hardness. Discrepancies detected through monitoring systems enable immediate intervention, preventing inconsistent outcomes and ensuring that the steel meets the required strength and hardness specifications.
- Material Characterization
Post-treatment analysis of the material is vital for verifying the effectiveness of the deep heat treatment and confirming that the desired properties have been achieved. Techniques like metallographic analysis, hardness testing, and tensile testing provide concrete evidence that the material properties meet the specified requirements. Comparing results to pre-defined benchmarks and specifications ensures that the process meets quality standards and that the material is suitable for its intended application. This approach, which is common in automotive manufacturing, confirms that components exhibiting the correct hardness and toughness are produced consistently, ensuring the safety and reliability of the vehicles in which they are used.
- Process Documentation and Audit Trails
Maintaining comprehensive documentation of the entire process is crucial for ensuring traceability and accountability. Detailed records of temperature profiles, time durations, and specific material characteristics are essential for process validation and for identifying any potential issues that may arise during the treatment process. Regular audits of these records, procedures, and equipment help ensure compliance with industry standards and regulatory requirements, further enhancing the reliability and safety of the process. In pharmaceutical manufacturing, documentation of every step of the sterilization process is imperative to ensure that the products are safe for human consumption. Audit trails verify that procedures are consistently followed and help trace any deviations or potential issues that may need to be addressed.
- Statistical Process Control (SPC)
Applying statistical methods to monitor process variations is a key element of quality assurance. SPC charts track key process variables, facilitating identification of trends or anomalies that may indicate potential problems. Early detection of deviations from the norm enables timely corrective actions, preventing costly issues that might arise from inconsistencies or deviations during deep heat treatments. This methodology, which is particularly important in manufacturing processes, helps ensure that the final product adheres to stringent quality standards and meets the required performance criteria. For instance, in a semiconductor fabrication plant, SPC helps manage temperature variations during diffusion processes, ensuring that the devices are manufactured with consistent and reliable electrical characteristics.
Comprehensive quality assurance in deep heat treatment provides confidence in the reliability and consistency of the final product, reducing the risk of failures and ensuring that the materials meet the required performance specifications. Implementing these procedures is essential for maintaining quality control, improving process efficiency, and safeguarding against potential production issues and safety risks. Consistent adherence to QA practices ensures the product's efficacy and reliability, directly impacting performance and longevity, which is particularly important in critical applications where failure is not an option. The robust standards employed in the QA process provide confidence in the material's performance and suitability for its intended use, enhancing the overall quality and reliability of the products in which these materials are used.
This section addresses common queries regarding deep heat treatment, providing concise and informative answers. The questions cover key aspects of the process, including technical details and practical implications.
Question 1: What distinguishes deep heat treatment from other thermal processes?
Deep heat treatment is distinguished by its focus on achieving specific, targeted material alterations through highly controlled temperature and time parameters. It differs from general heating processes by the intense precision in manipulating temperature gradients, heating and cooling rates, and the duration of exposure to elevated temperatures. This focused approach allows for precise control over the material's microstructure and resultant properties, leading to enhancements in strength, hardness, ductility, and other characteristics not typically achievable through simpler heating methods.
Question 2: What are the critical factors influencing the success of deep heat treatment?
Several factors influence the success of deep heat treatment. Material properties, such as thermal conductivity and chemical composition, dictate the optimal treatment parameters. Precise temperature control, encompassing careful monitoring of gradients and heating/cooling rates, is critical. Accurate time durations for specific temperature profiles are essential. Finally, the design of the treatment process itself plays a pivotal role in obtaining the desired outcome. Proper equipment calibration is also essential.
Question 3: What are the typical applications of deep heat treatment?
Deep heat treatment finds diverse applications across various industries. It's crucial in manufacturing high-performance alloys used in aerospace, automotive, and other industries that require components with enhanced strength and durability. Deep heat treatment is also employed in semiconductor manufacturing, food preservation, and other sectors requiring meticulously controlled material alteration for specific outcomes.
Question 4: What are the potential risks or limitations of deep heat treatment?
Potential risks include material distortion, unwanted phase transformations, and the development of internal stresses if parameters are not precisely controlled. Incorrectly calibrated equipment can lead to inaccurate temperature profiles, ultimately compromising the quality of the heat-treated material. Furthermore, some materials might exhibit reduced ductility or increased brittleness if subjected to inappropriate heat treatment conditions.
Question 5: How can the effectiveness of deep heat treatment be evaluated?
The effectiveness of deep heat treatment is evaluated through a variety of methods, including material analysis techniques (e.g., metallographic analysis), mechanical property testing (e.g., hardness testing, tensile testing), and visual inspection. These procedures verify if the material characteristics meet specified requirements and standards. Comparison to pre-treatment data provides a definitive measure of the treatment's success.
These FAQs provide a foundational understanding of deep heat treatment. Further research into specific applications or materials may offer more detailed insights.
- Breaking Darrell Crumps Net Worth Revealed And How He Made It
- Who Is Nicole Carter Carmellas Rise To Wwe Fame Amp More
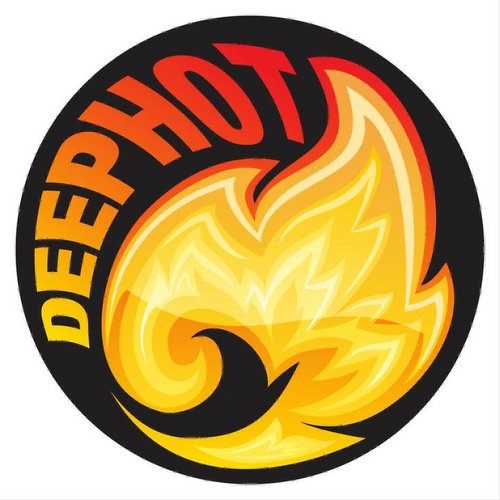
DEEPHOT RECORDS (DEEPHOTRECORDS) Twitter
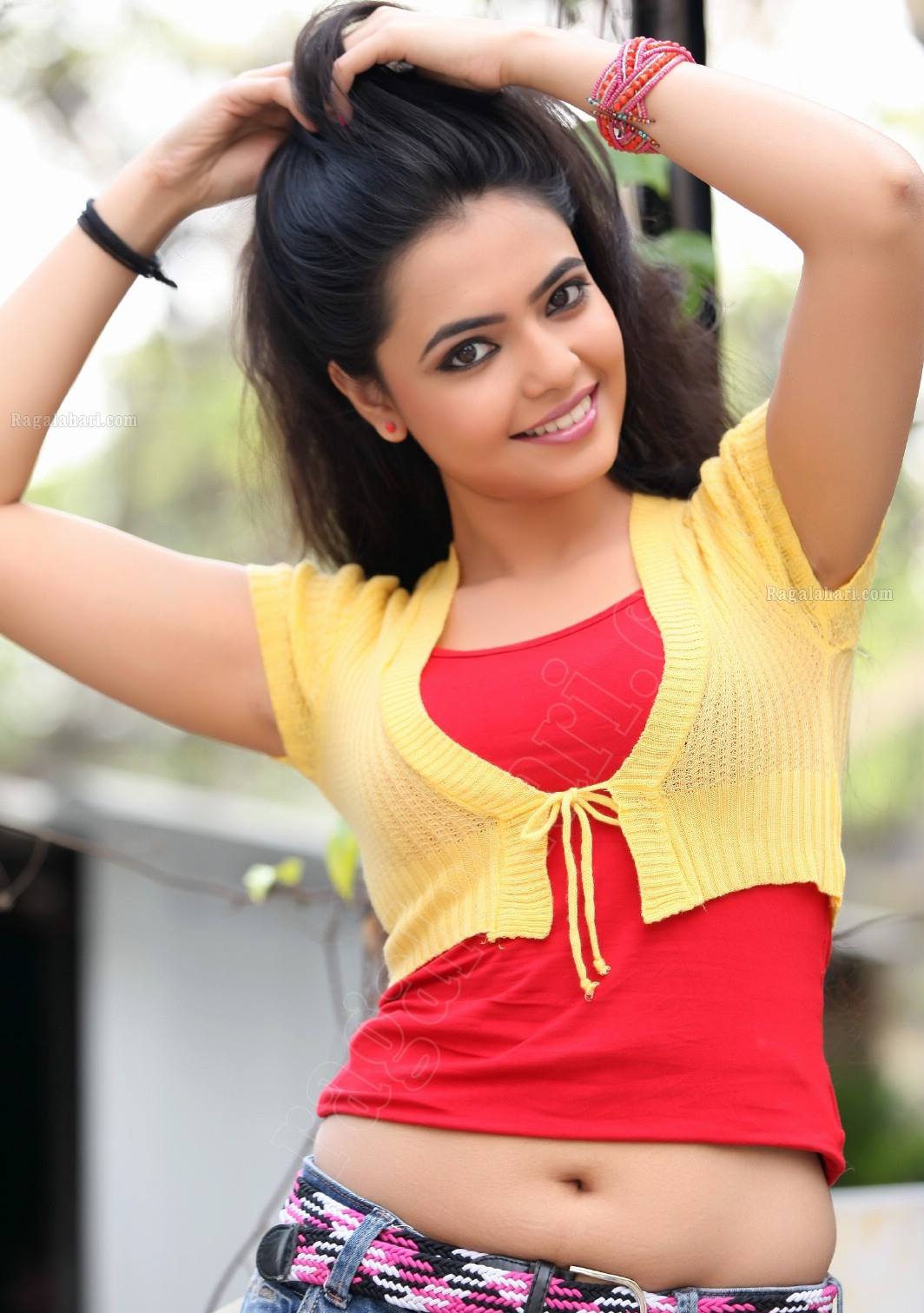
Tamil tv serial actress hot photoshoot lasopareader
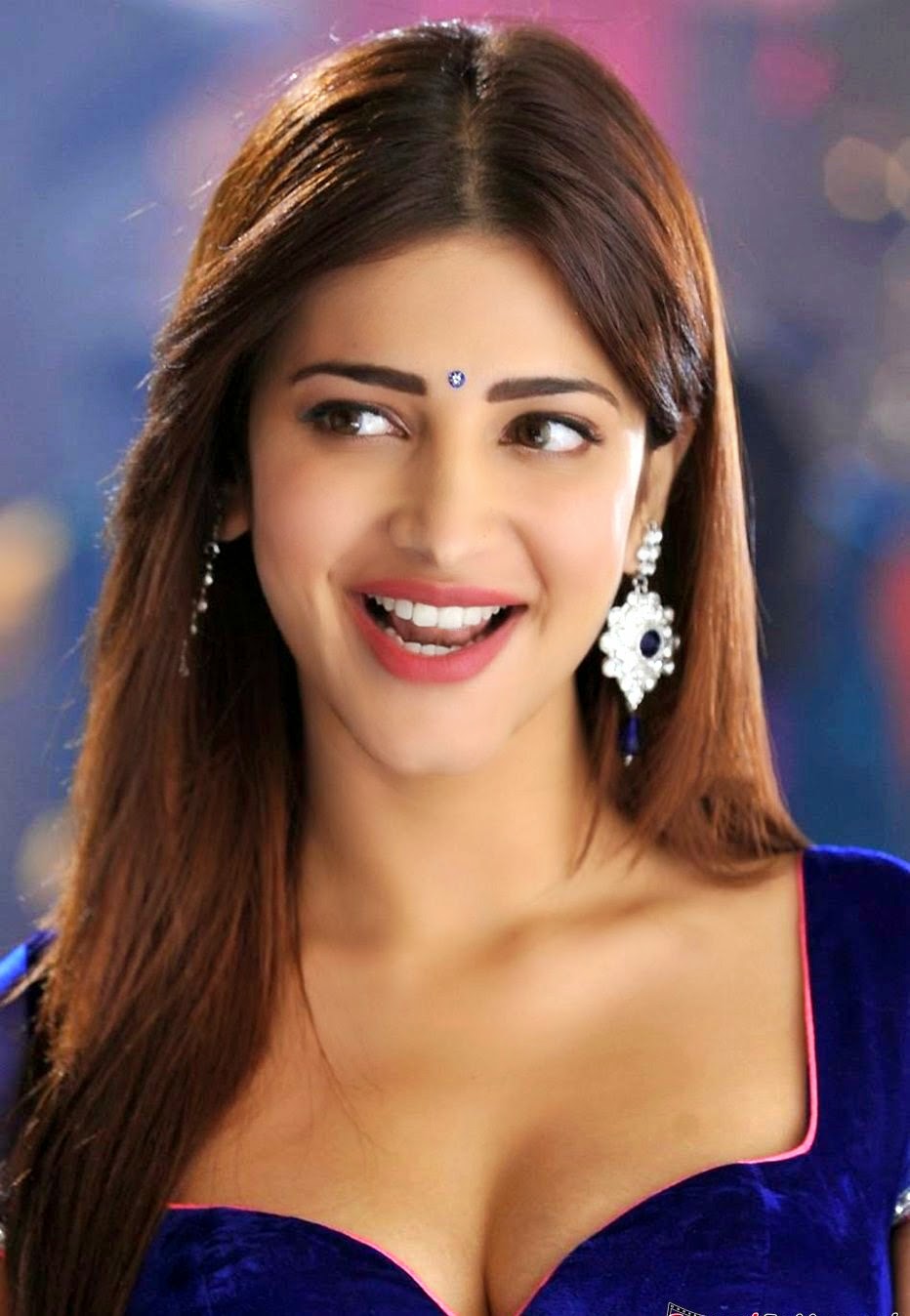
Katrina Kaif Shruti Deep Cleavage Show Pics 2014